Characterising sources of groundwater contamination
By W McCance, OAH Jones, A Surapaneni and M Currell.
First published in Water e-Journal Vol 5 No 2 2020.
Abstract
Assessing contamination impacts on groundwater resources to meet ongoing regulatory requirements can be difficult. This is particularly relevant for Wastewater Treatment Plants (WWTPs) that are often located in environments with multiple potential contamination sources.
Conventional monitoring methods are often unable to distinguish between site and off-site-derived contamination impacts, leading to uncertainties when determining compliance with site licence conditions.
This paper focusses on equipping site operators and their consultants with a range of novel groundwater tracers – specifically radioactive isotopes, stable isotopes and Contaminants of Emerging Concern (CECs) – to help address this challenge.
These tracers, when used in conjunction with routine monitoring methods, have shown great potential in better characterising wastewater derived impacts on underlying groundwater systems and distinguishing them from other sources in the catchment, such as agriculture.
This provides greater insight and certainty into the assessment of impacts to groundwater and a potential means of enhancing ongoing monitoring, management and/or remedial works.
Introduction
The potential for wastewater treatment plants (WWTPs) to cause adverse impacts to the surrounding environment is a major global environmental challenge (Bdour et al., 2009, Chitikela et al., 2012), with the prevention of significant local impacts on aquatic biota and water resources being a major regulatory focus.
However, despite the need to provide regulators with a definitive assessment of compliance in accordance with a site’s licence conditions and the relevant regulatory framework, assessing impacts on groundwater resources, which exist within the sub-surface, can be difficult.
This is particularly important for WWTPs, which often occur in urban, peri-urban or intensively farmed areas where multiple potential sources may contribute similar contaminants, leading to difficulties assessing and managing risks to the receiving environment (Robertson et al., 2013, Van Stempvoort et al., 2013).
There is a need for robust and sensitive techniques that can unravel different sources of groundwater impacts, and distinguish wastewater-derived contamination from other potential sources (Adebowale et al., 2019, McCance et al., 2018).
Such techniques can help to ensure the ongoing assessment and management of legacy and/or recent groundwater impacts is commensurate with the site’s contribution to contamination risks.
Techniques which can accurately identify and differentiate multiple contamination sources in the vicinity of WWTPs have the potential to save tens to hundreds of thousands of dollars in monitoring costs, and in the long-term help to minimise or better target potentially costly remedial actions.
Application and scope
The intent of this technical paper is to equip WWTP operators with knowledge of a set of novel groundwater tracers that can be used to more accurately assess and manage contamination risks specifically related to site activities.
Such risks may include leakage from historic unlined or partially lined treatment assets, damaged treatment assets, or other infrastructure involved in treatment and transporting wastewater and solid wastes, leaching from biosolids storage areas, use of treated effluents and/or accidental release of treated or partially treated effluents.
The application of these novel groundwater tracers can provide a more definitive approach to constraining wastewater-derived impacts on the groundwater environment as distinct from other similar sources
(i.e. wastewater derived nutrients versus agricultural-derived sources).
This tracer approach is intended to supplement conventional monitoring methods, whereby novel groundwater tracers are used in conjunction with conventional methods in order to provide greater clarity and understanding of the nature of impacts in complex environments.
It is noted that the use of novel groundwater tracers may not be warranted in all cases – e.g. where contamination sources are relatively well known and there are few additional sources of contamination in proximity to the site. This should be assessed in accordance with the guidance provided in this technical paper and other relevant sources (e.g. (McCance et al., 2018)).
Over time, it is expected that as the tracer methods described here are more widely applied, they should reduce operator costs through informing the design of more targeted monitoring, management and (potentially) remedial strategies, compared to what could be achieved via the use of conventional routine monitoring methods (e.g. major ions, nutrient concentrations, bacteriological indicators and physical parameters, etc.) alone.
Prerequisites
Prior to applying novel groundwater tracer methods, it is important to have a thorough understanding of existing site conditions, including hydrogeological characteristics, site history and potential sources of contamination – as outlined in Stages 1 and 2 of the site characterisation process (Figure 1).
Preliminary and detailed site investigations should be conducted in line with the National Environmental Protection (Assessment of Site Contamination) Measure (National Environment Protection Council, 1999).
The site investigation process will provide the foundations to progress to Stage 3 of the site characterisation process (assessment and delineation of contamination sources), shown in Figure 1.
As a minimum, Stage 1 and Stage 2 works should include:
- Completion of a Preliminary Site Investigation (PSI) in accordance with the NEPM Schedule B2 (National Environment Protection Council, 1999), targeting the historic land uses and potential on-site and off-site sources of contamination.
- Establishment of a suitable environmental monitoring network covering the potential sources of contamination and background conditions (e.g. groundwater, surface water and treated or partially treated effluent).
- Completion of a Detailed Site Investigation (DSI) in accordance with the NEPM Schedule B2 (National Environment Protection Council, 1999), targeting the potential sources of contamination and the nature/extent of contamination.
- Development of a detailed Conceptual Site Model (CSM).
Where Stage 1 and 2 investigations have identified the existence of potential wastewater-derived impacts on groundwater quality in mixed-source environments, the application of novel groundwater tracers may be considered as part of Stage 3 of the site characterisation process.
This is particularly relevant where conventional methods (e.g. routine water quality monitoring) have proven unsuccessful in adequately delineating the nature and extent of the contamination plume(s).
Novel groundwater tracer methodology
Radioactive isotopes
The use of naturally occurring radioactive isotopes in groundwater studies often relates to the determination
of groundwater ‘age’ or ‘residence time’ – which can be defined as the time spent in the aquifer since entering
the groundwater system (i.e. recharge). Over time,
these radioactive isotopes decay to a more stable configuration (Clark, 2015), enabling the determination of groundwater ‘age’.
As each radioactive isotope decays at a unique rate, the applicability of each radioactive isotope for ‘dating’ water following different flow paths depends on their approximate residence time range, determined from the radioactive half-life (Table 1).
The use of radioactive isotopes is particularly useful in identifying the flow patterns (i.e. groundwater mixing), assessing the renewability of groundwater resources (Clark, 2015), and assessing vulnerability of aquifers to contamination (Jasechko et al., 2017).
Analysis of multiple radioactive isotopes with different half-lives from the same location is also a powerful method for better understanding the extent of mixing between relatively ‘old’ groundwater – unlikely to have been affected by contamination in modern times – and ‘young’ groundwater which is more vulnerable to contamination during recharge from the surface (Jasechko et al., 2017).
In the context of assessing wastewater-derived contamination, radioactive isotopes can be used to
assist in:
- Identifying areas where potential leakage from treatment infrastructure may have impacted groundwater quality – e.g. indicated by the presence of ‘young’ groundwater (i.e. elevated activities of tritium and related isotopes with short half-lives).
- Determining the origin and timescale(s) of contamination (i.e. recent versus legacy) by using the spatial distribution of activities compared to the site development timeline.
This concept and rationale behind the use of radioactive isotopes has been depicted visually in Figure 2.
In combination with knowledge of a site’s history, the use of radioactive isotopes can assist WWTP operators in determining the residual risk profile of a site (e.g. identifying areas of recent or ongoing leakage of wastewater into the underlying groundwater system) and assist in determining suitable management and/or remedial actions to prevent impacts to surrounding beneficial uses.
Source: Cartwright et al., (2017) and Clark (2015)
Notes: Bold text – indicates common, readily available isotopes. The remaining isotopes presented are subject to limited availability and often focussed on research rather than commercial application.
In selecting the appropriate radioactive isotopes, it is important to have a thorough understanding of the CSM including:
- The flow regime (i.e. hydraulic conductivity and seepage velocity)
- Groundwater flow direction
- Site development timeline
Normally, more than one radioactive isotope should be sampled from each well to account for potential mixing – e.g. between ‘relatively old’ groundwater, and ‘young’ groundwater (which could be a result of recent contamination). Such mixing can create a ‘bi-modal’ residence time distribution, which can only be detected with the aid of multiple isotope tracers (Cartwright et al., 2017).
Stable isotopes
It has long been understood that in agricultural regions, groundwater quality can be impacted by multiple contaminants including fertilisers, animal manure or septic tank effluents (Clark and Fritz, 1997, Kendall and McDonnell, 1998).
This is particularly true in urban, peri-urban or intensively cultivated agricultural areas where it makes the identification of the origins and pathways of groundwater contamination – and hence, suitable management and/or remediation strategies – difficult.
One approach to distinguishing potential sources and mechanisms of contamination in these environments is the use of stable isotopes such as δ2HH2O, δ18OH2O, δ15NNO3, δ18ONO3 and δ13CDIC, which may be diagnostic of particular sources of contamination (Figure 3).
In assessing the isotopic composition and spatial variation of stable isotope signatures, it is possible to distinguish between two or more contributing pollution sources – provided these exhibit distinctive isotopic signatures.
In many contexts, the existence of unique isotopic signatures between different contamination sources (e.g. Figure 3) makes these promising tracers in delineating wastewater-derived contamination from
other sources.
In some cases, the use of stable isotopes may also be useful in estimating the contribution from different sources to the overall contaminant pool via the use of end member mixing calculations, which involve mixing two end-member components of different (and distinctive) chemical and isotopic composition (i.e. end-members) (Kendall and McDonnell, 1998).
The composition of the resulting mixture will vary depending on the relative abundance of the end members. It is therefore possible to determine the mixing relationship, and hence to estimate the contributions from various sources (taking into account possible secondary modification processes such as denitrification, which also affects nitrate isotopic signatures (Xue et al., 2009).
In the context of assessing wastewater-derived contamination, stable isotopes can be useful in constraining multiple sources of similar contamination (such as nutrients), enabling the separation of site-derived versus off-site impacts.
However, the effectiveness of applying stable isotope methods for contaminant source attribution are typically reliant on the occurrence of distinct end members between background groundwater and the specific source(s) of contamination.
This can be problematic in settings where multiple off-site inputs (e.g. related to fertiliser use, animal manure or septic tank effluents) or secondary modification of isotope signatures (e.g. through denitrification) preclude the identification of distinct background and source end member concentrations, leading to significant uncertainty (Clark and Fritz, 1997, Kendall and McDonnell, 1998, Wang et al., 2017).
Ultimately, the effectiveness of individual isotopic tracers is dependent on the variability of the isotopic signatures between end members and the subsequent mixing and fractionation behaviour in the subsurface environment.
As such, in some cases, combining isotopic data with hydrogeochemistry tools (such as major ion data) and other tracers (such as radioactive isotopes and CECs) may be required to more definitively separate different sources of contamination.
Contaminants of emerging concern
Recently, research into alternative groundwater tracers has identified the use of Contaminants of Emerging Concern (CECs) as a promising tool to identify wastewater-derived impacts as distinct from other potential sources of contamination (McCance et al., 2018).
CECs in this context include a range of persistent organic pollutants (POPs) such as pharmaceuticals and personal care products, artificial sweeteners, industrial compounds, and pesticides/herbicides.
Given wastewater influents and effluents are thought to be the main source of CECs in the global environment (Benotti et al., 2009, Lapworth et al., 2012), it can be expected that a range of CECs may be present in the sub-surface environment due to the infiltration of wastewater from legacy or recent infrastructure leaks and their incomplete removal during the wastewater treatment process.
Whereas, CECs such as certain pesticides and veterinary pharmaceutics are more likely to come from agricultural sources.
A recent assessment of potential novel groundwater tracers (McCance et al., 2018) identified a range of CECs, including persistent pharmaceuticals and personal care products (carbamazepine, crotamiton, primidone, atenolol and sulfamethoxazole), artificial sweeteners (acesulfame, sucralose, saccharin and cyclamate), and certain pesticides/herbicides (atrazine and simazine) as promising tracers for delineating wastewater impacts on groundwater systems.
This was based on the following four key selection criteria:
- Sufficient presence in wastewater influents and/or effluents and after treatment processes (where WWTP impacts are of interest); in order to be readily detected in contamination plumes.
- Diagnostic of WWTP impacts as opposed to other off-site potential contamination sources.
- Persistent in the sub-surface environment (i.e. resistant to biodegradation, sorption and transformation).
- Amenable to rapid and sensitive analysis.
In assessing the findings of CEC analysis in groundwater samples around a WWTP site, the spatial distribution of concentrations in relation to site infrastructure - which may be impacting groundwater, can first be analysed.
Secondly, relationships (i.e. ratios) of one compound to another – e.g. a specific CEC to a conventional indicator such as nitrate or ammonia, or one CEC to another – can be valuable in distinguishing wastewater-derived contamination from other potential impacts.
In some cases, this can also provide insight into historic and more recent contamination sources that may have differing source concentrations and degradation rates over time. Analysis of these data in conjunction with the radiogenic and stable isotopic data may then add a further layer of understanding.
Conceptual framework for use of novel groundwater tracers
To assist WWTP operators in assessing the potential selection and application of appropriate novel groundwater tracers for a given site, the following framework (Figure 4) is provided.
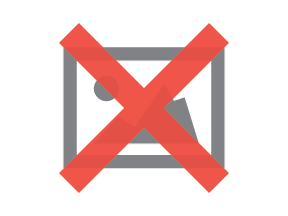
In mixed and/or multiple source environments, a combination of methods is likely to provide a more definitive assessment of wastewater-derived impacts and their relative contribution to the observed contamination.
When combined, these methods provide multiple lines of evidence to give greater certainty in delineating wastewater derived impacts, which can be of great value in working with environmental auditors and/or regulators to develop appropriate management strategies (Figure 5).
Case study
The use of novel groundwater tracers has recently been applied to a WWTP located in south-east Australia to assist in constraining the nature and extent of an identified nutrient plume, which led to the operator reporting a non-compliance in accordance with the licence conditions.
The plume (consisting predominantly of ammonia and nitrate) has been subject to routine groundwater monitoring since 1997, however, to date, conventional routine groundwater monitoring works have been unable to accurately distinguish site-derived impacts from other potential sources – such as the surrounding agricultural operations (predominantly horticulture).
This is further complicated by the complex site history, which indicated both historic impacts from unlined, partially lined or clay lined treatment assets and recent impacts from damaged treatment assets, despite routine monitoring works indicating the existence of only a single plume.
In this case, given the potential for impacts over multiple timescales, changes in water composition over time and the existence of CECs in plant effluent, a combination of novel groundwater tracers were applied to further constrain the wastewater-derived impacts and provide a greater understanding of the nature and extent of groundwater impacts. This is helping to inform future management and/or remedial actions at the site.
Due to the WWTP site being located within an area of significant agricultural activity and use of recycled water from the plant within the region, the first step in the process was to investigate the local and regional characteristics of the nutrient plume via the analysis of stable isotopes of nitrate.
Outcomes of this work indicated the presence of two clear sources; an up-gradient source related to the intensive market garden activity and an on-site source related to potential infiltration of raw or partially treated effluent (Adebowale et al., 2019).
However, the exact contribution and magnitude of these impacts could not be accurately determined due to the variation in isotopic signatures within each contaminant source and the complications introduced by potential denitrification processes.
Following this, additional investigations incorporating the use of radioactive isotopes, stable isotopes and CECs were undertaken at a site level to reduce data gaps and further establish contamination sources and mechanisms in line with the multiple lines of evidence approach
(Figure 5).
Based on the findings, the radioactive isotope tritium revealed that leakage from on-site treatment assets had resulted in the underlying groundwater system being contaminated with wastewater-derived nutrients since the early 1990s.
This was corroborated by water stable isotope results that exhibited an evaporation signature consistent with impacts from above-ground water storages (i.e. above ground treatment assets).
Taking this a step further, end member mixing calculations using the water stable isotope results indicated three main contributing factors:
- An on-site source (i.e. current treatment assets)
- A potential off-site source (i.e. surrounding market gardens)
- An intermediate source (i.e. former treatment assets)
The results from the tritium and water stable isotope investigations were then used in conjunction with CEC analysis, focussing on tracing select CEC compounds identified in site effluent throughout the groundwater system. The findings of this work revealed a complex series of ‘hot spots’ within the broader plume (Figure 6).
The findings more accurately reflect the various contributions and changes in treatment assets over time (as indicated by the PSI) and have led to the identification of two ‘hot spots’ reflecting historic impacts and one more recent ‘hot spot’ reflecting recent impacts from damaged treatment assets.
The use of CECs also confirmed the presence of some off-site impacts – related to the surrounding agricultural operations – however, the CEC data also revealed clear differences between the groundwater thought to be affected by the WWTP as opposed to off-site sources (i.e. surrounding agricultural operations).
This not only supports the radioactive and stable isotope results but provides greater insight into the residual risks associated with different inputs. The findings of this work can be used to enhance the site’s CSM and assist in targeting future monitoring, management and/or remedial actions.
Furthermore, the application of novel groundwater tracers, can be used to assist operators in assessing compliance or otherwise in accordance with their licence conditions.
Commercial availability and limitations
To assist WWTP operators in selecting and applying these methods, the following table outlines the potential analytes, typical cost range and turnaround times involved in the application of novel groundwater tracers. Refer to Appendix A for a list of commercially available laboratories and their capabilities.
It is noted that these costs could be further reduced on a site-by-site basis under industry research opportunities.
1. Turn around times can vary depending on the nature of the analysis. Commercial turnaround times can be significantly faster than academic pursuits.
2. Existing commercially available methods may not be suitable for groundwater analysis due to detection limits ranging from 10-500 ng/L. Refer to Table 3 for reported concentration ranges.
Effluent concentrations sourced from: (Buerge et al., 2009, Busetti et al., 2015, Cardenas et al., 2016, Dong et al., 2015, Kase et al., 2011, Kasprzyk-Hordern et al., 2009, Köck-Schulmeyer et al., 2013, Nelson et al., 2011, Oppenheimer et al., 2011, Subedi et al., 2015, Yang et al., 2011, Ying et al., 2009)
Groundwater Concentrations sourced from: (Cary et al., 2013, Edwards et al., 2019, Fenz et al., 2005, Kahle et al., 2009, Köck-Schulmeyer et al., 2014, Koroša et al., 2016, Kuroda et al., 2012, Nödler et al., 2013, Sui et al., 2015, Van Stempvoort et al., 2011, Wolf et al., 2012)
Conclusion
The use of novel groundwater tracers or co-tracers, whilst still in its infancy, provides a means that not only assists in discriminating between up-gradient and site-derived impacts, but also provides insights into historic and more recent contamination sources and their changing characteristics.
This can play an integral role in enhancing the outcomes of routine monitoring works and providing a greater understanding of the nature and extent of groundwater impacts in mixed source environments, enabling ongoing monitoring, management and remedial actions to be suitably targeted.
This can enable WWTP operators to accurately determine compliance or otherwise in accordance with the site’s licence and the relevant regulatory framework.
Despite the current limitations in terms of access to these methods, a small number of providers already analyse a range of these tracers (i.e. stable and radioactive isotopes) on a commercial basis.
The outcomes can be used to supplement existing datasets and provide greater insight into the origins and pathways of contamination, as well as provide, a basis for enhancing monitoring programs, informing management or remedial actions, or refining remedial goals.
The lack of access to commercial CEC analysis at sufficient sensitivity for typical concentrations observed in groundwater at this point in time creates some barriers to adoption. However, given most commercial laboratories do have the instrumentation required to undertake analysis, there is the possibility of expanding their capabilities in response to the needs of the market.
Acknowledgements
The authors would like to thank CRC CARE, Gippsland Water, Melbourne Water, South East Water, and Western Water for their funding and support in undertaking this work, without which this would not be possible.
About the authors
William McCance | Higher Degree by Research - PhD Candidate, RMIT University
Will graduated from RMIT University in 2013 where he completed a double Bachelor of Environmental Science and Bachelor of Engineering (Environmental). After completing his degree, Will worked as a contaminated land consultant for several years before returning to RMIT to undertake his PhD, focussing on the identification and application of novel groundwater tracers in delineating wastewater impacts. His research interests include water science and technology, hydrogeology and contaminated land management.
Professor Oliver A.H Jones | Associate Dean - Biosciences and Food Technology, RMIT University
Oliver received his MSc and PhD from Imperial College London in 2005. Prior to joining RMIT he worked as a postdoctoral fellow at the University of Cambridge and then a lecturer at the University of Durham. His research interests include water science and technology and the trace analysis of environmental pollutants and the assessment of their effects in biological systems.
Dr. Aravind Surapaneni | Senior Research Scientist and Planning, South East Water
Aravind received his PhD degree from Massey University, New Zealand in 1994. After his studies, he began working for the Department of Primary Industries at Tatura (near Shepparton in Victoria) and for a pharma industry in the U.S. before moving to the water industry in 2009. His research interests focus around the application of industry-based research as well as providing biosolids, soils and groundwater expertise to South East Water that meet the needs of the business.
Associate Professor Matthew Currell | Associate Dean – School of Engineer, RMIT University
Matt received his PhD from Monash University in 2011. His research focusses on using environmental isotopes and other tracers to understand groundwater sustainability and quality issues in diverse settings around the world.
Appendix A – Commercially available laboratories and capabilities
Notes:
* Indicates turnaround time has been estimated
NA – Not Available
Pricing and turnaround times are indicative only and may be subject to additional fees (e.g. shipping, batch fees, etc.)
References
ADEBOWALE, T., SURAPANENI, A., FAULKNER, D., MCCANCE, W., WANG, S. & CURRELL, M. 2019. Delineation of contaminant sources and denitrification using isotopes of nitrate near a wastewater treatment plant in peri-urban settings. Science of The Total Environment, 651, 2701-2711.
BDOUR, A. N., HAMDI, M. R. & TARAWNEH, Z. 2009. Perspectives on sustainable wastewater treatment technologies and reuse options in the urban areas of the Mediterranean region. Desalination, 237, 162-174.
BENOTTI, M., TRENHOLM, R., VANDERFORD, B., HOLADY, J., STANFORD, B. & SNYDER, S. 2009. Pharmaceuticals and Endocrine Disrupting Compounds in U.S. Drinking Water. Environmental Science & Technology, 43, 597.
BUERGE, I. J., BUSER, H.-R., KAHLE, M., MÜLLER, M. D. & POIGER, T. 2009. Ubiquitous occurrence of the artificial sweetener acesulfame in the aquatic environment: an ideal chemical marker of domestic wastewater in groundwater. Environmental Science & Technology, 43, 4381.
BUSETTI, F., RUFF, M. & LINGE, K. L. 2015. Target screening of chemicals of concern in recycled water. Environmental Science: Water Research & Technology, 1, 659-667.
CARDENAS, M. A. R., ALI, I., LAI, F. Y., DAWES, L., THIER, R. & RAJAPAKSE, J. 2016. Removal of micropollutants through a biological wastewater treatment plant in a subtropical climate, Queensland-Australia. Journal of Environmental Health Science & Engineering, 14, 14.
CARTWRIGHT, I., CENDÓN, D., CURRELL, M. & MEREDITH, K. 2017. A review of radioactive isotopes and other residence time tracers in understanding groundwater recharge: Possibilities, challenges, and limitations. Journal of Hydrology, 555, 797-811.
CARY, L., CASANOVA, J., GAALOUL, N. & GUERROT, C. 2013. Combining boron isotopes and carbamazepine to trace sewage in salinized groundwater: A case study in Cap Bon, Tunisia. Applied Geochemistry, 34, 126-139.
CHITIKELA, S. R., SIMERL, J. J. & RITTER, W. F. 2012. Municipal Wastewater Treatment Operations—The Environmental and Energy Requirements. World Environmental And Water Resources Congress 2012. Reston, VA: American Society of Civil Engineers.
CLARK, I. D. 2015. Groundwater geochemistry and isotopes, Boca Raton, CRC Press.
CLARK, I. D. & FRITZ, P. 1997. Environmental Isotopes in Hydrogeology, Florida, CRC Press LLC.
DONG, M. M., TRENHOLM, R. & ROSARIO-ORTIZ, F. L. 2015. Photochemical degradation of atenolol, carbamazepine, meprobamate, phenytoin and primidone in wastewater effluents. Journal of Hazardous Materials, 282, 216-223.
EDWARDS, Q. A., SULTANA, T., KULIKOV, S. M., GARNER-O'NEALE, L. D. & METCALFE, C. D. 2019. Micropollutants related to human activity in groundwater resources in Barbados, West Indies. Science of the Total Environment, 671, 76-82.
FENZ, R., BLASCHKE, A. P., CLARA, M., KROISS, H., MASCHER, D. & ZESSNER, M. 2005. Quantification of sewer exfiltration using the anti-epileptic drug carbamazepine as marker species for wastewater. Water Science & Technology, 52, 209.
JASECHKO, S., PERRONE, D., BEFUS KEVIN, M., BAYANI CARDENAS, M., FERGUSON, G., GLEESON, T., LUIJENDIJK, E., MCDONNELL, J., J, TAYLOR, R., G, WADA, Y. & KIRCHNER, J., W 2017. Global aquifers dominated by fossil groundwaters but wells vulnerable to modern contamination. Nature Geoscience, 10.
KAHLE, M., BUERGE, I. J., MÜLLER, M. D. & POIGER, T. 2009. Hydrophilic anthropogenic markers for quantification of wastewater contamination in ground‐and surface WATERS. Environmental Toxicology and Chemistry, 28, 2528-2536.
KASE, R., EGGEN, R. I. L., JUNGHANS, M., GÖTZ, C. & JULIANE, H. 2011. Waste Water - Evaluation and Management, InTech.
KASPRZYK-HORDERN, B., DINSDALE, R. M. & GUWY, A. J. 2009. The removal of pharmaceuticals, personal care products, endocrine disruptors and illicit drugs during wastewater treatment and its impact on the quality of receiving waters. Water Research, 43, 363-380.
KENDALL, C. & MCDONNELL, J. 1998. Isotope Tracers in Catchment Hydrogeology, Amsterdam, Elsevier Science.
KÖCK-SCHULMEYER, M., GINEBREDA, A., POSTIGO, C., GARRIDO, T., FRAILE, J., LÓPEZ DE ALDA, M. & BARCELÓ, D. 2014. Four-year advanced monitoring program of polar pesticides in groundwater of Catalonia (NE-Spain). Science of the Total Environment, 470-471, 1087-1098.
KÖCK-SCHULMEYER, M., VILLAGRASA, M., LÓPEZ DE ALDA, M., CÉSPEDES-SÁNCHEZ, R., VENTURA, F. & BARCELÓ, D. 2013. Occurrence and behavior of pesticides in wastewater treatment plants and their environmental impact. Science of the Total Environment, 458, 466-476.
KOROŠA, A., AUERSPERGER, P. & MALI, N. 2016. Determination of micro-organic contaminants in groundwater (Maribor, Slovenia). Science of the Total Environment, 571, 1419-1431.
KURODA, K., MURAKAMI, M., OGUMA, K., MURAMATSU, Y., TAKADA, H. & TAKIZAWA, S. 2012. Assessment of groundwater pollution in Tokyo using PPCPs as sewage markers. Environmental Science & Technology, 46, 1455.
LAPWORTH, D. J., BARAN, N., STUART, M. E. & WARD, R. S. 2012. Emerging organic contaminants in groundwater: A review of sources, fate and occurrence. Environmental Pollution, 163, 287-303.
MCCANCE, W., JONES, O. A. H., EDWARDS, M., SURAPANENI, A., CHADALAVADA, S. & CURRELL, M. 2018. Contaminants of Emerging Concern as novel groundwater tracers for delineating wastewater impacts in urban and peri-urban areas. Water Research, 146, 118-133.
NATIONAL ENVIRONMENT PROTECTION COUNCIL 1999. National Environmental Protection Measure.
NELSON, E. D., DO, H., LEWIS, R. S. & CARR, S. A. 2011. Diurnal variability of pharmaceutical, personal care product, estrogen and alkylphenol concentrations in effluent from a tertiary wastewater treatment facility. Environmental Science & Technology, 45, 1228.
NÖDLER, K., HILLEBRAND, O., IDZIK, K., STRATHMANN, M., SCHIPERSKI, F., ZIRLEWAGEN, J. & LICHA, T. 2013. Occurrence and fate of the angiotensin II receptor antagonist transformation product valsartan acid in the water cycle-a comparative study with selected β-blockers and the persistent anthropogenic wastewater indicators carbamazepine and acesulfame. Water Research, 47, 6650.
OPPENHEIMER, J., EATON, A., BADRUZZAMAN, M., HAGHANI, A. W. & JACANGELO, J. G. 2011. Occurrence and suitability of sucralose as an indicator compound of wastewater loading to surface waters in urbanized regions. Water Research, 45, 4019-4027.
ROBERTSON, W., VAN STEMPVOORT, D., SOLOMON, D., HOMEWOOD, J., BROWN, S., SPOELSTRA, J. & SCHIFF, S. 2013. Persistence of artificial sweeteners in a 15-year-old septic system plume. Journal of Hydrology, 477, 43.
SUBEDI, B., CODRU, N., DZIEWULSKI, D. M., WILSON, L. R., XUE, J., YUN, S., BRAUN-HOWLAND, E., MINIHANE, C. & KANNAN, K. 2015. A pilot study on the assessment of trace organic contaminants including pharmaceuticals and personal care products from on-site wastewater treatment systems along Skaneateles Lake in New York State, USA. Water Research, 72, 28-39.
SUI, Q., CAO, X., LU, S., ZHAO, W., QIU, Z. & YU, G. 2015. Occurrence, sources and fate of pharmaceuticals and personal care products in the groundwater: A review. Emerging Contaminants, 1, 14-24.
VAN STEMPVOORT, D., ROY, J., BROWN, S. & BICKERTON, G. 2011. Artificial sweeteners as potential tracers in groundwater in urban environments. Journal of Hydrology, 401, 126-133.
VAN STEMPVOORT, D., ROY, J., GRABUSKI, J., BROWN, S., BICKERTON, G. & SVERKO, E. 2013. An artificial sweetener and pharmaceutical compounds as co-tracers of urban wastewater in groundwater. Science of the Total Environment, 461-462, 348-359.
WANG, S., ZHENG, W., CURRELL, M., YANG, Y., ZHAO, H. & LV, M. 2017. Relationship between land-use and sources and fate of nitrate in groundwater in a typical recharge area of the North China Plain. Science of the Total Environment, 609, 607-620.
WOLF, L., ZWIENER, C. & ZEMANN, M. 2012. Tracking artificial sweeteners and pharmaceuticals introduced into urban groundwater by leaking sewer networks. Science of the Total Environment, 430, 8-19.
XUE, D., BOTTE, J., DE BAETS, B., ACCOE, F., NESTLER, A., TAYLOR, P., VAN CLEEMPUT, O., BERGLUND, M. & BOECKX, P. 2009. Present limitations and future prospects of stable isotope methods for nitrate source identification in surface- and groundwater. Water Research, 43, 1159-1170.
YANG, X., FLOWERS, R. C., WEINBERG, H. S. & SINGER, P. C. 2011. Occurrence and removal of pharmaceuticals and personal care products (PPCPs) in an advanced wastewater reclamation plant. Water Research, 45, 5218-5228.
YING, G.-G., KOOKANA, R. S. & KOLPIN, D. W. 2009. Occurrence and removal of pharmaceutically active compounds in sewage treatment plants with different technologies. Journal of Environmental Monitoring, 11, 1498-1505.