Analysing water sensitive urban design options
By X Meng and S Kenway.
First published in Water e-Journal Vol 3 No 4 2018.
Abstract
Planning for ‘water-sensitive’ infill development has become a priority for sustainable urban development in Australia. With city growth, infill development in vacant or under-used land parcels is becoming a dominant mode of development. However, the current ‘knock-down-rebuild’ approach to such development will undermine resource efficiency, liveability and urban amenity, particularly for natural water systems. There is a need to identify development options that are more water sensitive. To better analyse water efficiency and hydrological performance of Water Sensitive Urban Design (WSUD) options, we use a water mass balance evaluation framework to demonstrate the ‘whole of water cycle’ approach. The new insights gained were the extent to which the proposed WSUD options impact the water metabolism performance of the urban area through modelling the water mass balance of eight selected WSUD options within different scenarios, involving pre-development (1825), current (2016), before and after water-sensitive options in 2031. The novel contribution is the development of one new hydrological performance indicator, open space ratio and redeveloped other four water metabolism indicators to assess water performance in infill development. Results show that urbanisation dramatically altered the water flows, like stormwater runoff. Also, road space plays an important role in future solutions.
Introduction
Growing population and urbanisation change water flows due to the creation of impervious hard surfaces, removal of endemic plants, changes in land use, alteration of water use and introduction of water-based recreation (Cosgrove & Rijsberman, 2014; Loucks & Beek, 2017). These activities lead to a massive and comprehensive change of the hydrological system across a range of spatial scales. For example, in most of the watershed studies, stormwater runoff and sedimentation rapidly increase with urbanisation (Meyer & Turner, 1992; Booth & Jackson, 2007; Novotny, et al., 2010). Increased stormwater runoff has direct effect on a range of issues, such as a crisis of water quality (Astaraie-Imani, et al., 2012), sedimentation and erosion issues (Nie, et al., 2011), risk of flooding (Wahl & Plant, 2015; Raadgever & Hegger, 2018), waterborne diseases (Hunter, et al., 2001), pollution of underground water (Lenny, et al., 2011), aquatic species issues (Quattro, et al., 2002) and acidification of water bodies (Grunewald & Schoenheinz, 2014).
To alleviate the pressures from these ‘business-as-usual’ (BAU) developments, in many existing urban areas, particularly those where rainfall is extremely uneven, development with water sensitive urban planning and resources management has been introduced (Queensland Government, 2017). In fact, developing water-sensitive designs for infill development is an ‘urgent task’ for South East Queensland (SEQ) as larger numbers of redevelopment projects are currently using a ‘knock-down-rebuild’ approach (Queensland Government, 2017). To better manage WSUD options, we look forward to a new ‘whole of water cycle’ approach to assess the hydrological performance of infill development in the case study area, Norman Creek catchment. This new way for water evaluation may assist stormwater management approaches in Australia, and particularly Brisbane.
Water sensitive urban design (WSUD)
WSUD options include sustainable technologies in water management; new technologies in the anthropogenic hydrological flow recycling; new architectural designs in land use; and new designs in natural hydrological flow harvesting (Payne, et al., 2015). Rainwater collection and reuse is recognised as one of the most effective ways to reduce stormwater runoff and pollution at an affordable cost (Imteaz, et al., 2011). Similarly, wastewater recycling is another pathway to reduce the wastewater discharge. Additionally, with the development of new sustainable technologies, many WSUD projects can be applied in city development to alter the impervious fraction through new architectural designs, such as green roofs, green fences, horticulture gardens, shared gardens and linear parks (Engelman, 2013; Payne, et al., 2015).
Overall, these WSUD applications can: reduce the volume and peak flow; increase evapotranspiration and infiltration; decrease imperviousness ratio; improve stormwater runoff quality; convert some pollutants into inert substances; add to neighbourhood aesthetics; improve land value; recover biodiversity; and supply an alternative and local water source (Payne, et al., 2015). This paper will examine eight different WSUD options in a semi-hypothetical catchment-scale urban area – Norman Creek, Brisbane.
Urban mass balance and UMEF4Water
An urban water mass balance is a comprehensive account involving all water inputs, outputs, and storages, such as precipitation, centralised supply, evapotranspiration, stormwater runoff, wastewater discharge, groundwater infiltration and storage (Kenway, et al., 2011). Moreover, Kenway, et al. (2011) pioneered the use of urban water mass balance for metabolism evaluation by using it to evaluate a city’s water characteristics. Researchers have since applied this innovative approach in other case studies (Marteleira, et al., 2014; Farooqui, et al., 2016).
In fact, there are two different opinions in the water mass balance establishment; the first approach can be applied to water supply infrastructure to inform design so that supply matches demand (Bach, et al., 2014). The second concentrates on hydrological catchments to understand the influence of urbanisation on natural hydrological flow (Haase, 2009). Based on these two opinions, Kenway, et al. (2011) developed the urban metabolism system to study ‘anthropogenic’ hydrological and ‘natural’ flows at the same time, and also highlighted that water resources management needs to treat ‘cities as catchments’.
Additionally, Urban Metabolism Evaluation Framework for Water (UMEF4Water) is a new framework system to assess hydrological performance in an urban area as a whole, rather than individual components of the water budget within an urban area. Generally, there are four steps in this evaluation framework, involving: defining the urban boundary; collecting data about water inflows and outflows; generating a water mass balance; and assessing water metabolic performance of an urban area through indicators (Renouf, et al., 2016).
Method
To test the utility of the urban water metabolism evaluation framework in the infill development, in this paper we will: 1) model water performance (water mass balance) with different WSUD scenarios; and 2) assess the hydrological performance of each option and analyse how they mitigate high volume runoff and spatial variation. This will involve:
- Defining WSUD scenarios (Table 1).
- Generating a water mass balance.
- Assessing water metabolic performance through
We selected Norman Creek catchment (South East Queensland, Australia) as the study area. The catchment was surveyed and named by Edmond Lockyer in 1825, and at that time was almost impenetrable (BCC, 2010a). This catchment has an area of about 3,038ha, runs through 23 suburbs and contains 90,000 residents (BCC, 2010b). Urbanisation has reshaped the land use and the relationship between residents and the local waterways, causing high pollutant runoff, overland flow flooding, water shortages and reduced liveability.
In this project, the hydrological flows in the Norman Creek catchment under different scenarios were calculated based on the rainfall-runoff modelling algorithm of MUSIC (Model for Urban Stormwater Improvement Conceptualisation) (Water by Design, 2010; CRCWSC, 2018), which is a model commonly used for stormwater infrastructure design (Elliott & Trowsdale, 2007). Cadastral and land use information about the Norman Creek catchment was collected from the previous research including thirteen subsystems and roof, car park, driveway, amenity area, open space, and road for four selected subsystems (Appendix A and B). We hypothesised that WSUD projects may influence the hydrological performance of the whole catchment due to change of imperviousness for each subsystem.
Defining WSUD scenarios
The Norman Creek catchment has high volume runoff and spatial variation. In response to these issues, this catchment requires appropriate water-sensitive designs to restore water flows. This will involve an assessment of:
- Planning guidelines: Both state planning policies (Shaping SEQ Strategy) and Brisbane City Council (BCC) planning scheme support the application of water sensitive approaches to future infill development, Brisbane City Sharp 2026 (2006) highlighted that green roofs, shared and community gardens, wastewater recycling, stormwater collection from the road will be encouraged to be implemented (Queensland Government, 2017).
- Technical evaluation: An evaluation of design options to determine a solution that will increase evapotranspiration and groundwater infiltration; decrease stormwater runoff; and reduce the potable water demand in the city (Engelman, 2013; Payne, et al., 2015).
- Past examples of WSUD cases in Australia: Horticulture and shared gardens applied in Brisbane (Guitart, et al., 2014); green roof cases implemented in Melbourne and Adelaide (Victorian Government, 2014); grasscrete has been applied in Brisbane and many areas in Australia (BCC, 2006).
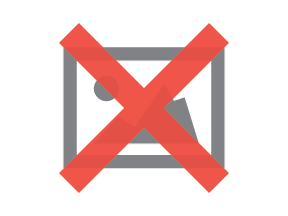
Assumptions of WSUD options
For this study, we have adopted two WSUD scenarios, a conservative implementation and a maximised implementation case as well as the business as usual (BAU) scenario (Figure 1). The details are set out in Table 1.
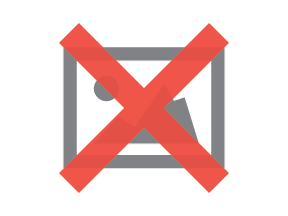
Generate water mass balance
After having selected the design scenarios, the second step is to generate a water mass balance for each scenario, the pre-development year 1825, the current year 2016, future year (BAU) 2031, and hypothetical water sensitive interventions in 2031. Water mass balance is an equation used to describe the water flows into and out of the system. From a hydrological perspective, it represents the sum of water inflows equalling water outflows and the change in storage. To address limitations in the traditional balance equation, there are some new water flows to be identified, such as water from recycling and precipitation harvesting.
The water metabolism evaluation framework (Figure 2) is developed from the water mass balance, treating an urban area as a whole (Kenway, et al., 2011). The water system developed for this work quantified ‘anthropogenic’ fluxes, including decentralised supplies of harvested precipitation (D), centralised supply (C), wastewater discharge (W), greywater recycling (R) and ‘natural’ flows, involving precipitation (P), groundwater infiltration (GI), evapotranspiration (ET) and stormwater runoff (Rs) (refer to Equation 1).
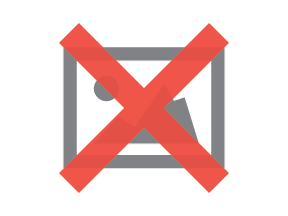
Equation 1: Input and output about water account
Input (Qi) = Output (Qo) + ΔS
Input (Qi) = (P’ + C + D + R) and Output (Qo) + ΔS = (ET + Rs + W+ GI + R) + ΔS
Where:
P=precipitation falling (P’ = unharvested precipitation)
C=centralised water supplies sourced from outside the urban boundary, which could include surface waters and groundwater
D=decentralised water supplies harvested inside the urban boundary, including rainwater, stormwater and groundwater
ET=evapotranspiration from the study site boundary
Rs=stormwater run-off discharged from the study area boundary (not including that which is harvested within the urban boundary)
W=wastewater discharged from the urban boundary (total wastewater generated less that which is recycled internally or externally)
GI=groundwater infiltration
R=reuse/recycling of wastewater within the urban boundary
ΔS=change in the stored water volume within the defined boundary
In this research paper, the after-development introduces two water flows to the pre-development water system, including centralised water supply (C) and wastewater discharge (W). After that, there are two new flows that are added to the refined model. One is rainwater harvesting (D) from WSUD Option 1 rainwater reuse; precipitation (P) in this study is separated into unharvested precipitation (P’) and rainwater harvesting (D). The other flow is greywater recycling (W) from WSUD Option 2 greywater recycling, which is flowing out of the system and re-entering after treatment as a new input. Around 70% of wastewater is greywater (Farooqui, et al., 2016).
Assess water metabolic performance
The last step of water evaluation framework in infill development is to assess the water metabolism performance through UMEF4Water indicators. Based on the previous water metabolism studies from Renouf et al. (2016), we had developed a total of eight water metabolism indicators to assess the hydrological performance of infill development options at the study site. In Table 2, these indicators are categorised into two groups: resource efficiency and hydrological performance.
Indicators of resource efficiency
Water extracted is an existing UMEF4Water indicator, concerning water efficiency which is used to assess water usage before and after water-sensitive options. Some researchers define the water efficiency to include both direct and indirect use, where direct use considers the local environment only, while indirect use involves global supplies (Huang, et al., 2013). In this study, water efficiency focuses on direct water used locally and uses indicator ‘water extracted’ to represent the overall efficiency of water. The second and third indicators from UMEF4Water are used to evaluate harvested stormwater and recycled wastewater in this work. They are internal harvesting ratio and internal recycling ratio respectively.
WSUD indicators of hydrological performance
The last is a set of five indicators, four of them are redeveloped from previous UMEF4Water research, including stormwater runoff, total stream discharge, infiltration, and evapotranspiration ratio (Farooqui, et al., 2016). The fifth is a new indicator, ‘open space ratio’. These five indicators for the first time are used to assess infill development projects for water resources protection and hydrological flows restoration (Table 2). Moreover, the open space ratio is a practicable indicator introduced to assess water restoring in infill development, because it is readily available with access to data concerning open space.
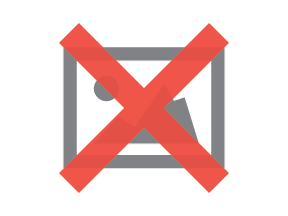
C=centralised water supply, D=decentralised water supply, R=reuse of wastewater, Rs=stormwater runoff
Results and discussion
The results from the following Table 3 and two figures (Figure 3 and 4) show that BAU will maintain the study site as a ‘typical’ infill development scenario, with higher imperviousness, more runoff, less evapotranspiration and less infiltration, compared to current hydrological performance in the Norman Creek. Moreover, the benefit is that the WSUD can help alleviate negative impacts of typical infill development, and especially demonstrate that:
- Hydrology and stormwater runoff can change due to development;
- Road space is a large part of the future solution; and
- How the ‘whole of water cycle’ method is applied in the infill development context.
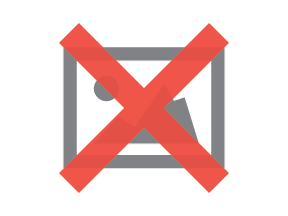
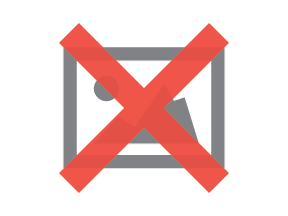
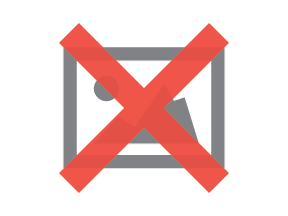
is 2031 BAU7
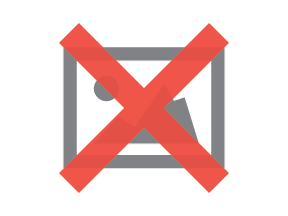
3.1 Hydrology and stormwater runoff change due to development
Although multiple factors have a mixed effect on hydrological flows, the overall impact of urbanisation typically leads to hydrology change (Feng, et al., 2016). According to the results, stormwater runoff and total stream discharge are now 384% and 497% greater than the pre-development case (1825) (Table 4). Also, for an average rainfall year (2016), groundwater infiltration (GI), evapotranspiration (ET) and open space (OS) decreased by 31%, 38% and 27% respectively from pre-development (Figure 4), and a huge increase of stormwater runoff by 17.5GL/yr (from pre-development 6.2GL/yr to current 23.7GL/yr), which are similar to another case study in SEQ (McIntosh, et al., 2013).
Compared to the pre-development case (1825), the present (2016) total water input has increased by 7.5 GL/yr and will increase by further 1.3 GL/yr by 2031 (Table 4). This means that there will be more water demand in the future if there are no attempts to restore natural hydrology. It can also be noted that TSD will increase 537% compared to the pre-development case, which is 40% more than in 2016 (Table 4). There will be more stormwater runoff, less evapotranspiration and less groundwater infiltration for BAU in 2031 relative to pre-development in 1825.
To compare and assess hydrological performance about selected WSUD options, Table 3 details the water mass balance for each option, along with the water metabolism indicators quantified using the mass balance in 2031 and compares eight WSUD options in two different implementation scenarios (the base case 2031 BAU). The results clearly show that stormwater runoff increased over 282% in an average precipitation year due to the uneven rainfall and greater impervious surfaces after urban development. Figure 5 shows how the different individual WSUD options and how the aggregrated options in the maximised case will influence runoff. The results show that:
- Urban development is dramatically changing the hydrology in the Norman Creek catchment.
- The aggregation of all WSUD options has the best water performance in all options.
- For all individual WSUD options, the option with the most potential to influence stormwater flows is the linear park.
Overall, the hydrological performance will deteriorate unless high imperviousness is mitigated, and pre-development hydrological conditions are restored. Compared to pre-development, this research paper predicted around 17.6 GL/yr stormwater runoff may run across the land after rain if it can not enter the stormwater drainage system in 2031 (Figure 5). To alleviate this, WSUD projects need to be introduced. Among all WSUD options in Table 3, the linear park from Option 8 with maximised implementation has the best performance to decrease stormwater runoff. The linear park also has the best hydrological performances on imperviousness, ET and GI.
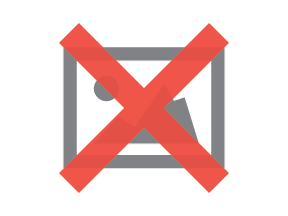
Clarify how road space is a large part of the future solution
This project observed that WSUD options will impact the runoff through land use heterogeneity in the study area, due to:
- The higher road and intersection areas which form part of the overland flow system. This is around 22% (668 ha) of surface land in the study site used for transportation.
- The higher density of impervious land will cause more stormwater discharge to storm sewer systems(Gallo, et al., 2013).
- The high density of impervious channels will decrease the stream channel storage and at the same time, groundwater infiltration will decrease(Gallo, et al., 2013).
As shown in Table 3 and Table 5, urban planners and water resources managers can shift the overall impervious fraction in an urban subsystem (high imperviousness ratio) to a more water-sensitive urban area (low imperviousness ratio) through the WSUD options implementation. Especially, the linear park option in the maximised case can help the Norman Creek catchment shift the imperviousness ratio for the whole catchment by 5.8% (decrease from 67.2% to 61.4%), meaning over 200 ha road and intersection area (high impervious fraction) becomes linear park (low impervious fraction). In addition, the linear park option can decrease runoff by over 1.4GL/yr, compared to the current year (2016).
Obviously, it is an opportunity for city planners to add more water-sensitive designs, such as the linear park in new urban planning projects to improve hydrological performance through impervious fraction change.
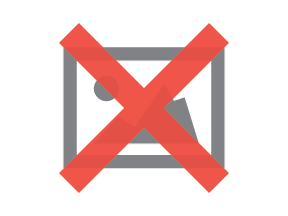
The utility of a ‘whole of water cycle’ method applied in the infill development context
This research paper is the first time to use the ‘whole of water cycle’ method in the infill development context, also the first time to test UMEF4Water evaluation framework within WSUD development, including to define WSUD scenarios, generate a water mass balance and assess hydrological performance.
To better assess water performance in UMEF4Water, we redevelop four water metabolism indicators (stormwater runoff ratio; total stream discharge ratio; infiltration ratio and evapotranspiration ratio) and introduce a new indicator (open space ratio) within the infill development context. In this case, there are eight different WSUD options to be selected to fit the different subsystems and we assume all subsystems can supply a maximum of land use transfers from high imperviousness towards natural land use status.
UMEF4Water can also be used to assess water sustainability, resources management and greater liveability in the urban environment. The next stage of the research needs to focus on how to develop more appropriate UMEF4Water indicators, particularly, to evaluate water, water-related energy, and material performance. The appropriate UMEF4Water indicators associated with water, energy and material can aid urban planners in evaluating metabolism performance with a broader view. Also, the completely established evaluation framework can support the City Plan to assess infill development projects precisely and rapidly.
Conclusion
This research paper demonstrates how a ‘water metabolism’ perspective can be used to generate water efficiency and performance indicators to assist water resource management in the study area due to the high demand for WSUD. Based on results, WSUD options from this project such as linear parks should be applied to more infill development projects for better hydrological performance.
In this work, WSUD options have been analysed for future urban development. New insights include:
- Urban development has a dramatic alteration for the hydrological system of Norman Creek.
- The implementation of selected WSUD options has a benefit on both water efficiency and natural water flows restoration; they are proposed to mitigate stormwater runoff impacts. Of all the options evaluated, the linear park option has been shown to provide the greatest benefit of restoring natural hydrological flows.
- Introducing more UMEF4Water indicators for infill development.
Wider use of UMEF4Water system and indicators based on this work would help urban planners and water managers to identify more water-sensitive options for infill development. This would likely have an alternative systematic analysis effect on urban design and water management. It would also help quantify hydrologically efficient and water-sensitive performance in the future.
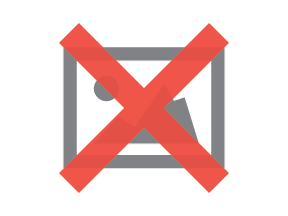
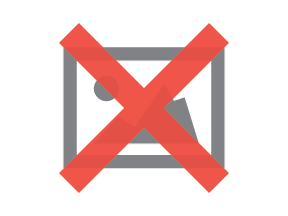
Acknowledgements
This work was supported by the Brisbane City Council (BCC), International WaterCentre (IWC) and Cooperative Research Centre for Water Sensitive Cities (CRC WCS) IRP4, which is funded by the Australian Government and industry partners. We would especially like to thank the input of Dr Ka Leung Lam and Dr Marguerite Renouf from the Water-Energy-Carbon Research Group, The University of Queensland who provided technical guidance.
About the authors
Xuli Meng
Xuli is a dynamic, highly motivated water specialist with a desire to influence the development of water sensitive urban planning. He started as a Civil Engineer from the industry. There he gained highly experience in civil and environmental engineering. Focused on using his knowledge and experience to effect real global change, Xuli came to Australia in 2016 to participate in the IWC’s Master of Integrated Water Management (University of Queensland), where he pursued an urban-focused specialisation in Water Sensitive Urban Design (WSUD). After graduation, Xuli joined Natural Resources Access Regulator (NRAR), New South Wales Government in 2018.
Associate Professor Steven Kenway
Dr Kenway is a scientist with 28 years experience in academia, CSIRO, industry, government and consulting. His research focusses on urban water and wider metabolism assessment and management. He creates quantitative analytical tools for understanding and managing water and related energy, GHG emissions, food, and cost flows through utilities, households, cities, and economies. This involves developing conceptual frameworks, analysis methods and partnerships addressing direct and embodied impacts of water supply and use.