Developing novel sodium-ion batteries for sewage pumping stations
By Jonathan Knott, Danny Sutanto, Kashem M. Muttaqi, Duane Robinson, Sean Elphick, Shi Xue Dou, Heriberto Bustamante and Ruben Muller.
First published as an Ozwater'18 Conference Paper.
Abstract
Sewage pumping stations are vital to water utility operations, ensuring the removal of wastewater from customer areas to treatment facilities for processing. This paper describes the motivation, methodology and results for characterisation of the energy requirements of a sewage pumping station during operation, and the process to determine how a renewable energy generation and storage system can maximise self-generation/consumption and increase energy resilience for a selected Sydney Water sewage pumping station.
The work presented here has been undertaken as part of the Smart Sodium Storage Solution (S4) Project – a $10.5m, Australian Renewable Energy Agency-funded international consortium project to develop novel, low-cost sodium-ion battery technology for renewable energy storage, and to demonstrate this technology in several applications. The thorough characterisation of this end-use application is critical to ensure the sodium-ion battery cells and modules are designed to be fit-for-purpose, and will be able to supply the energy and power requirements of the Sewage Pumping Station.
Introduction
Pumping both fresh and waste water throughout water utility networks is an energy-intensive activity, and accounts for almost 41% of the approximately 400 GWh of energy used by Sydney Water each year [Sydney Water Corporation (2016)]. The rapid decline in cost of renewable energy generation and storage technologies – particularly solar PV and battery-based storage [Bloomberg Finance L.P. (2017)] – has opened many new opportunities for implementing these technologies to reduce grid energy consumption and increase energy resilience.
Sydney Water, as part of their Energy Strategic Plan, has identified sewage pumping stations (SPSs) as an area of operations that require a holistic energy management solution to increase energy resilience. Sewage pumping stations are a major component of the operations of Sydney Water – which has 685 SPSs throughout its network – that ensure the safe and efficient transport of waste water from communities to treatment plants for processing.
Most SPSs operate intermittently as part of a large, interconnected network, and they must be available under all external operating conditions. It is particularly important for Sydney Water to ensure SPS operation can occur during extreme events such as bushfires (which are prevalent in the outer areas of their network), adverse weather conditions, and are able to operate during electricity supply grid failure. The implementation of local renewable energy generation and energy storage at SPS sites may be a viable solution to provide this capability. This solution has a secondary benefit of addressing safety risks and costs associated with emergency repairs to the grid or implementation of backup generators to maintain SPS operation during grid outages. As such, there are two energy-focused modes of operation for a renewable energy generation and storage system relevant to SPS applications – an energy resiliency mode to maintain operations during extreme conditions, and self-generation/consumption maximisation mode to reduce electricity costs during standard operational conditions.
A comprehensive understanding of the energy and power requirements of an SPS is crucial to developing a battery-based renewable energy generation and storage system that is fit-for-purpose and fulfils the operational needs of the SPS. The key parameters required to ensure such a system is appropriate are the peak and continuous power, and the daily energy consumption. In addition, the power quality disturbance levels for equipment at the SPS site should be determined to ensure they fall within acceptable limits and will not interfere with operation of the SPS.
This paper describes the methodology employed to characterise the electrical performance of SP0055, a Sydney Water sewage pumping station with an attached public amenities block located adjacent to Bondi Beach (Figure 1). This SPS was selected as a testbed for a renewable energy generation and novel sodium-ion battery-based energy storage system due to its representative nature of many of Sydney Water’s SPS assets.
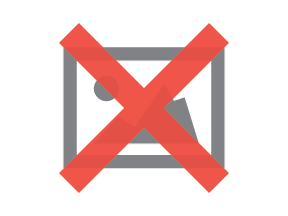
Experiment
Several complementary approaches were employed to characterise the SPS plant, power quality disturbance and overall power and energy operational requirements. Results from these activities were collated and cross-referenced to ensure congruence across the data acquisition methods.
Site survey
A comprehensive site survey was performed to determine the plant and ancillary equipment located at the SP0055 site. SP0055 was constructed in 1927 and is a “dry well”-type design. This design features an outer wet well that collects incoming sewage from surrounding homes and commercial buildings and an inner dry well that houses the main pumps. A level sensor engages the pump when the level in the sump reaches a predetermined value, and a second level sensor shuts off the pump when the sewage level falls below the pre-set minimum level. A general layout of the SPS configuration is shown in Figure 2.
SP0055 contains plant including two main 6.9 kW positive displacement pumps with soft starters, a 1.1 kW sump pump with a direct-on-line (DOL) starter, a 1.5 kW ventilation fan, site lights and power outlets, and a SCADA control and monitoring system.
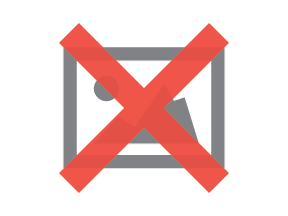
Electrical schematics of the site were obtained and were verified as accurate through site inspection. A simplified schematic of the plant installed at SP0055 is shown in Figure 3.
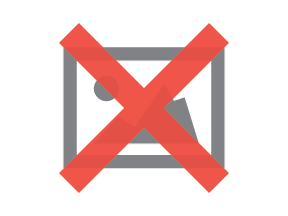
SCADA data acquisition
Sydney Water has a SCADA system installed across all remote sites to allow for monitoring and control of plant and equipment. This system is a Schneider-based SCADA system, and can access 30-minute power and energy data from the utility meter installed at SP0055. Additional information available from the SCADA system included details of pump on/off status.
High-speed data acquisition
A Hioki PW3198 Power Quality Analyzer [Hioki (2012)] was temporarily installed at SP0055 to capture power quality disturbances, pump start-up transients, and high-accuracy daily energy consumption data. This unit was installed as depicted in Figure 3. As the site is low voltage (230 V line-neutral), voltage was measured directly by the instrument. Current was measured using 100 A clamp-on current transducers.
Discussion and results analysis
The S4 Project has four main deliverables:
- A commercialisation-ready sodium-ion battery (SIB) chemistry and architecture that is low-cost and easily manufactured
- A modular and expandable packaging system for the sodium-ion battery cells, with integrated energy management system (EMS) including intelligent algorithms to establish the optimal generation, storage and consumption of energy at the site
- The demonstration of the sodium-ion battery modules and EMS at Sydney Water’s SP0055
- A sodium-ion battery manufacturing capacity and capability at the industry partner sites
SIBs have a number of advantages that makes them a viable alternative to lithium-ion batteries in applications where the small size and weight penalty of using sodium-ion batteries is not a significant issue. Most importantly, the raw materials for manufacturing SIBs are significantly cheaper than those for lithium-ion batteries (LIBs), and SIBs don’t require expensive and damaging-to-mine cobalt or nickel. The cell architecture, and hence manufacturing process, for SIBs is substantially similar to LIBs. This means the plant and expertise developed for manufacturing LIBs can be applied to SIBs, which is important for demonstrating the viability of SIBs as an alternative technology to LIBs.
The S4 Project is targeting deploying SIBs in renewable energy storage applications, such as SP0055, and it is critical to understand the application requirements so that the battery architecture, models and EMS can be tailored to meet operational needs. The comprehensive characterisation of SP0055 presented here provides key data used in shaping the design and development of the first three S4 Project deliverables.
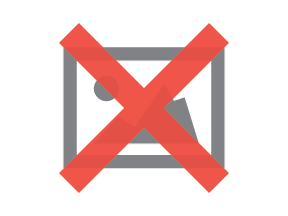
Half-hourly data for 1826 days of operation, from 1/9/12 to 31/8/17, was obtained from the energy meter system installed at SP0055, and was used to generate long-term trend analysis of site energy consumption. This was supplemented with high-speed data from the Hioki PW3198 collected over the period 11/10/17 to 16/10/17.
Daily energy consumption
The anticipated daily energy consumption is critical to assess when designing a renewable energy generation and storage system, as the assumptions used for estimating the daily energy consumption can have a significant impact on the calculated assets required to appropriately service the operational requirements. Figure 4 shows the daily cumulative energy consumption for the 1826 days of data from the Sydney Water SCADA system. The total daily energy consumption for each day is presented in the histogram in Figure 5, which shows a significant clustering around energy consumption of 7-8 kWh-per-day. Statistical analysis reveals that for 95% of the days analysed SP0055 had an energy consumption of 13.95 kWh-per-day or less, and 50% of the days had an energy consumption of 7.98 kWh-per-day or less.
Factors affecting daily energy consumption
An investigation was undertaken to determine if the energy consumption of SP0055 rose during operating conditions that include inclement weather events. If the energy requirement was correlated to weather events, it necessitates the use of higher percentile energy consumption figures to calculate energy storage requirements to ensure the system retains enough energy to allow SP0055 to operate in the energy resilience mode, due to the fact that local generation from solar PV is generally curtailed during inclement weather and electricity grid supply is at its least reliable. Figure 6 shows the daily energy consumption for SP0055 and the daily rainfall as reported by the Bureau of Meteorology’s (BOM) Rose Bay Automatic Weather Station (AWS), approximately 1.7km from SP0055 [Bureau of Meteorology (2017)].
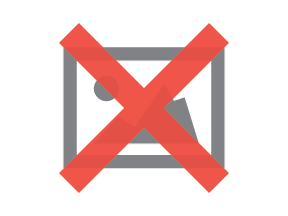
From Figure 6, the peak energy consumption for SP0055 clusters around the summer months, which is consistent with additional load being placed on the system by the adjoining public amenities block due to increased utilisation of Bondi Beach. This coincides with the peak daily insolation of the site as shown in Figure 7 data from the BOM Rose Bay AWS. This is a desirable correlation, as the pumping demand increases in concert with an increase in capacity for solar PV generation.
Instantaneous power consumption
A simplistic method of estimating the power draw of the system would be to sum the nameplate power requirements of all the plant in SP0055 and use that as the design parameter. This, however, would lead to a substantial overestimation of the likely power draw, which in turn would result in an expensive, over-specified and underutilised renewable energy generation and storage system. To develop a more realistic profile for the operation power requirements of SP0055, the control strategies for the site pumps were obtained from the Sydney Water network operations department. As noted in the Experiment section, the primary loads at the SP0055 site are the two main 6.9 kW pumps; however these pumps are configured to only operate one-at-a-time. This control scheme was confirmed by analysing the SCADA system data on the main pumps on/off status.
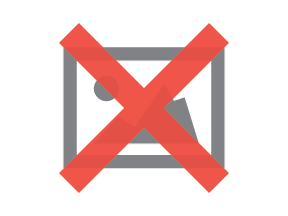
The maximum instantaneous peak power identified from the Hioki dataset was 23 kW total during pump start-up, settling to a continuous power draw of 9.5 kW total during pump operation. Figure 8 shows the transient behaviour of the start of one of the main pumps. No transients for the equipment with DOL starters (sump pump and ventilation fan) were recorded in the high-speed data analysis period; however the power draw profiles for these starters are well understood. A heuristic for characterising DOL starters is that they typically draw 6-8 times the rated current as the attached motor spins up to operating speed. As such, it is likely the components with DOL starters will have similar peak transient magnitudes as the main pumps.
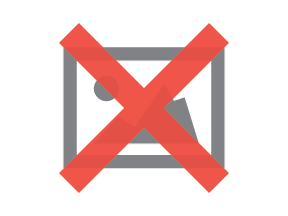
The maximum average half-hourly power recorded by the electricity meter was 4.31 kW (Figure 9). This is consistent with the meter operating at a lower measurement frequency, and averaging the power consumption over a longer period compared to the Hioki apparatus. This is also consistent with the pumps operating on a very low duty cycle, as running one of the main pumps for 15 mins would result in a half-hour average power of approximately 4.5 kW.
The instantaneous peak power and continuous power requirements of a system are critical in specifying components such as PV and storage inverters, and in ensuring the energy storage system is not damaged during transient events. In addition, if incorrectly specified the inverters or battery management system could shut down to prevent damage if higher than rated power is drawn – which would be a failure of the system design.
Factors affecting power consumption
A key factor affecting the overall site power draw is the concurrent operation of the plant. As can be seen in Figure 3, there is the potential for a large power draw if all plant is used concurrently, however even a smaller, unanticipated load can drastically alter the power draw of the system. The large daily energy consumption spikes present in the data in Figure 6 are primarily due to on-site work that included the use of power tools being undertaken. Addressing this level of uncertainty in the power draw of the site is critical when designing a renewable energy generation and storage system to meet the needs of the site.
Renewable energy generation and storage system
The in-depth system and historical data analysis presented here can be used to develop a specification for a renewable energy generation and storage system that meets the energy and power requirements of the SP0055 site.
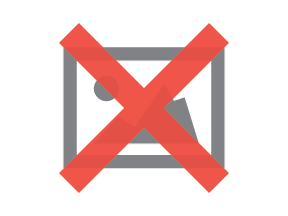
A key consideration for such a system is the primary purpose for installing the renewable generation and storage capability. There are a number of operational modes that can be used, including: peak shifting, self-generation/consumption, uninterruptable power supply, arbitrage, frequency and ancillary services, and demand management. Each of these modes has specific generation and storage requirements and uses the available resources in specific ways to optimise the mode performance.
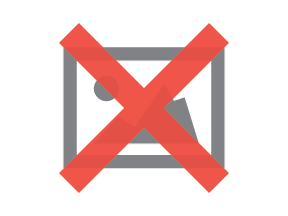
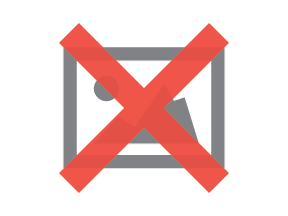
Energy security and resilience, and self-generation/consumption have been identified as the key modes of operation required for SP0055. This allows for design decisions tailoring the system configuration and composition to these specific modes to be made, resulting in a more efficient and cost-effective system.
The limiting factor for a renewable energy generation and storage system for SP0055 is the space available for the installation of solar PV panels. SP0055 is located on a public promenade, and as such the only available space to install solar PV panels is on the SP0055 roof (at other sites it may be possible to install free-standing solar PV panels adjacent to the SPS if there is available land). Figure 10 shows the approximate size of the roof area, including obstructions present. The available roof area has been calculated at approximately 35 m2 when accounting for all obstructions and access requirements. This results in a maximum possible generation capacity of approximately 7 kW, using 340 W PV panels at an optimal tilt angle.
However local council regulations for the area in which SP0055 is located limit the tilt angle to less than half the optimal value, which would reduce the overall generation capacity. From the data presented in Figure 7, this would result in a maximum theoretical energy generation of 63.61 kWh on the sunniest summer day, and 1.69 kWh under the most severe cloudy/rainy conditions. From the data analysed, there were three days in the week where the generation capacity would have been lower than the operational needs. This, however, is an outlier scenario in the five years of data analysed. Thorough risk analysis would need to be undertaken before configuring SP0055 in fully-islanded mode of operation (i.e. completely off-grid) as the minimum daily generation capacity is significantly lower than the average daily energy requirements. In a fully off-grid configuration, the connected energy storage would need to be sufficient to cover the shortfall in energy from the generation system, and would need to include sufficient reserve to maintain energy supply over several days of reduced generation capacity.
The theoretical maximum generation capacity is also appropriate for operating as a self-generation/consumption system, whereby the SP0055 site would be preferentially powered by energy generated by the installed solar PV, secondarily from on-site energy storage that is charged by the solar PV, and by the grid only when neither of these sources is available. This mode of operation would allow for significant reduction in grid electricity consumption, and therefore a reduction in the electricity cost for the site. For this mode of operation only, an energy storage capacity of 10 kWh has been estimated as appropriate to maximise performance and minimise capital cost.
As noted in the introduction, energy resilience is a critical target mode of operation for a renewable energy generation and storage system at SP0055, and one that can be achieved through the appropriate selection of inverters and energy storage assets. Most solar PV and energy storage inverters are grid-tied only, meaning they only operate when a grid electrical supply is present. This is to minimise the risk of local islanding, where in the event of a grid outage the PV/energy storage system could feed energy back into the local network, thus re-energising the system and creating safety hazards. Selecting inverters and protection equipment that can safely operate in islanded mode would allow for uninterrupted SP0055 operation in the event of a grid outage, thus providing energy resilience.
For the SP0055 site, a grid outage is not a common occurrence (there were no outages recorded in the 5 years of data analysed) and the average length of blackouts recorded across Australia and New Zealand in 2016 was 99 minutes [Eaton (2016)]. As such, an additional 7 kWh of energy storage would provide a minimum reserve capacity to supply the maximum power needs for the site for the average power outage duration. The actual capacity selected for this mode of operation above 7 kWh is therefore a risk-based calculation and is dependent on many factors including: the likelihood of a prolonged outage, the energy requirements during such an outage, the anticipated energy generation capability during an outage, the cost/time-frame for bringing an external generator to site, the storage time available in the SP0055 well, and many others.
The two modes of operation can be achieved concurrently through the use of intelligent control algorithms to apportion different amounts of the available energy storage pool for self-generation/consumption and energy resiliency applications. This “value stacking” is one of the key advantages of renewable energy generation and storage systems and provides compelling technical and financial advantages for a wide range of applications.
Impact on the design and development of novel sodium-ion batteries and modules
SP0055 has been selected as the primary testbed and demonstrator site for the sodium-ion batteries developed in the S4 project, and as such the application requirements are being used to inform the design and optimisation of the individual sodium-ion battery cells (shown in Figure 11), the battery modules, and the overall Energy Management System (EMS) being developed to manage and optimise energy generation, storage and consumption at the site.
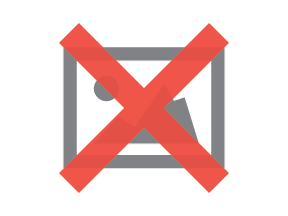
The usage profile data presented in this paper shows that the overall sodium-ion battery storage solution will need to have an approximate power-to-energy ratio of approximately 1.65 (23 kW / 13.95 kWh), which is significantly higher than most commercially-available energy storage solutions are designed for (the Tesla Powerwall 2 has a power-to-energy ratio of 0.52, the LGChem RESU10 a ratio of 0.71) [Solar Choice (2018)]. This ratio requirement has resulted in a design decision of implementing several smaller sodium-ion battery modules in parallel, rather than a single, large module. This has the added advantage of allowing the smaller modules to be designed with a power-to-energy ratio closer to 0.5, which will maximise their suitability for other applications.
The practical application requirements provides clear guidance for the development of the sodium-ion battery modules, with design decisions such as module IP rating, communication protocols, size, weight and mounting preferences all influenced by the specific needs of the system.
In addition, detailed characterisation of the SP0055 operational requirements also provides guidance to the development of the sodium-ion battery cells, as parameters such as charge/discharge rate, cycle life, voltage window, cell format and battery architecture. For example, the relatively high charge/discharge rate required to meet the peak power draw needs of the system has led to the focus on specific sodium-ion battery cathode materials that have excellent high-rate performance [Li et al. (2017), Deng et al. (2017)].
Conclusion
The development of a renewable energy generation and storage system for an SPS application requires careful and comprehensive consideration of the energy and power requirements for all anticipated operating conditions. This is particularly important for designing a system to provide energy security and resiliency through islanded operation in periods of grid failure. The framework for characterising a SPS is outlined in this paper, and the framework was used to characterise SP0055, a Sydney Water SPS located behind Bondi Beach.
Historical power and energy data for the site was analysed and showed variability in the operational power and energy requirements – with the daily energy requirement ranging from 5.01 kWh-per-day to 39.29 kWh-per-day. The power requirements of the site were assessed and the peak power drawn during main pump start-up was found to be 23 kW, with a continuous power draw of approximately 9.5 kW during operation.
This comprehensive analysis is discussed in this paper in terms of the requirements of a renewable energy-based system that could meet the operational requirements of SP0055, and has been used as the basis for developing a renewable energy generation and sodium-ion battery-based storage system to be installed at SP0055. Two usage scenarios for the SP0055 system were discussed, a mode maximising self-generation/consumption, and a mode where full off-grid operation would be possible. The storage requirements for each mode of operation were outlined, including the practical considerations around the risk of exhausting stored energy in the off-grid mode. Further details of the simulation and operational behaviour of this system will be presented in subsequent publications.
This study is fundamental to the design of the novel sodium-ion batteries that are being developed to meet the pumping station’s energy requirements. These batteries, coupled with the wider energy management system also under development, will provide a compelling demonstration of next-generation energy storage technology, and provide valuable insights and data for further rolling-out of such deployments at suitable SPS sites across Sydney Water’s network.
Acknowledgement
This project received funding from ARENA as part of ARENA’s Research and Development Programme. The S4 Project consortium consists of the University of Wollongong, Hebei ANZ New Energy Technology Co. Ltd, McNair Technology Co. Ltd, Liao Ning Hong Cheng Electric Power Co. Ltd, and Sydney Water Corporation.
Ozwater is the Australian Water Association's annual international water conference and exhibition which takes place in alternating cities each May. To find out more, visit the Ozwater website.