Alternative cyanobacteria management approaches
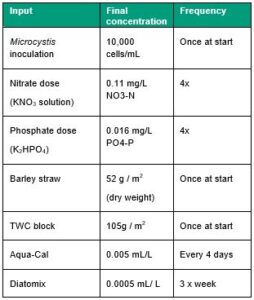
A Rohlfs, A Davie, J Pera
First published in Water e-Journal Vol 6 No 2 2021.
Abstract
Aeration is conventionally used to prevent cyanobacteria blooms in source water storages but is often prohibitively expensive. There are several treatment products on the market that may offer an alternative approach to cyanobacteria bloom prevention by manipulating the microbial ecology of a lake. WaterNSW recently tested selected treatment products in a 2-week laboratory study and a 10-week field study. The trials assessed how well the products controlled cyanobacterial growth, and their potential for adverse effects on water quality, treatability and ecological health. The laboratory trials provided some evidence to support the effectiveness and mode of action for specific treatments. However, the field trial found that destratification still had the strongest influence on cyanobacterial growth relative to other tested treatments. The trials provided many useful learnings regarding the effectiveness and safety of the treatment products, the circumstances where specific treatments might best be suited for deployment and the design of any future trials. The outcomes of this work will be used to evaluate the potential of these products for future use in managing cyanobacterial blooms in WaterNSW’s supply storages.
Introduction
Current climate models predict that in the coming decades NSW will become hotter, with longer and more intense droughts and more variable rainfall. These conditions favour the growth of harmful cyanobacteria which can make water unsafe to drink and have serious social, economic and ecological consequences.
Cyanobacteria blooms can be prevented through careful management of source water storages. Aeration is one such technique that prevents blooms by maintaining an unfavourable environment for cyanobacteria, but is often prohibitively expensive. An alternative approach may be to manipulate the microbial ecology of a lake to inhibit the formation of harmful cyanobacteria blooms. There are several emerging cyanobacteria treatment products on the market that are designed to do this. At present these products are primarily applied in aquaculture, wastewater management or for aesthetic purposes. While these treatments offer several potential benefits, there is limited information available on the efficacy of these treatments in larger drinking water supply storages. Furthermore, these treatments may pose risks to water quality, lake ecology and raw water treatability.
A preliminary review of cyanobacterial treatment products was undertaken, and four treatments were selected for trials after considering cost, risk to drinking water quality and potential for adverse ecological impacts. The shortlisted products included;
- Aqua-Cal+™
- Diatomix
- The Water Cleanser Block
- Barley straw
Aqua-Cal+™ (Calix) is a magnesium hydroxide slurry that binds dissolved nitrogen (N) and phosphorus (P) (Wu et al., 2001), reducing the nutrients available for cyanobacterial uptake. Diatomix (AlgaEnviro) is a micronutrient solution designed to promote the growth of diatoms, which can outcompete cyanobacteria under certain environmental conditions (Horn and Uhlmann, 1995, Patrick et al., 1969). The Water Cleanser (TWC) is a hydrocarbon block infused with trace elements that can be metabolised by Bacillus bacteria. Some strains of Bacillus sp. produce extracellular compounds that rupture cyanobacterial cells (Sigee et al., 1999, Wright and Thompson, 1985, Yu et al., 2015). Barley straw (Hordeum vulgare) has been applied as floating straw bales to treat cyanobacteria (e.g. Ball et al., 2001, Barrett et al., 1999). The mechanism for growth inhibition is thought to involve the release of phytotoxic phenolic compounds as the straw decomposes (Everall and Lees, 1997).
Two trials were undertaken to test the cyanobacteria treatments. An initial laboratory trial was carried out to assess short-term treatment effectiveness at controlling cyanobacterial growth and to identify any potential adverse effects on water quality, water treatability and ecological health. This was followed by a field trial to assess the effectiveness of barley straw and the TWC block relative to aeration, and to investigate the influence of environmental factors on observed cyanobacterial growth.
Method
Laboratory Trial
The laboratory trial included five treatment groups; Aqua-Cal+™, Diatomix, TWC, barley straw that had been pre-soaked for one week and a control. Each group contained four replicate 20 L glass tanks filled with water collected from the surface of Lake Prospect near WaterNSW monitoring site RPR6 (-33.827686, 150.884321) on 13/08/2019. The lake water was transported to the laboratory and dispensed into the incubation tanks within 6 hours of collection. Tanks were fitted with clear plastic lids, aerated with aquarium air stones and incubated at 27° under growth lamps on a 12-hr light/dark cycle. The tanks were spiked with a fresh, non-toxic Microcystis aeruginosa culture and with nutrient solutions as per Table 1. Baseline sampling was carried out on 14/08/2019 (Day 0). Treatments were applied immediately following the Day 0 sampling as per Table 1.
Physical and chemical variables were measured daily with an EXO2 multiprobe sonde (Xylem). Tank water grab samples were collected for nutrients, organic carbon and algae on Day 0 (14/8), Day 1 (15/8), Day 5 (19/8), Day 9 (23/8) and Day 14 (28/8). Nutrient and carbon concentrations were processed following standard analytical methods (Rice et al. 2017). Algal samples were analysed by microscopic identification and enumeration (Rice et al. 2017). Ecotoxicological assays were performed to assess the acute response of rainbow fish (Melanotaenia duboulayi), water fleas (Daphnia magna) and bacterial luminescence (Vibrio fischeri) to the treatments. Total and dissolved metal concentrations and total petroleum hydrocarbons (TPH) were measured with organic gas chromatography (OGC-FID). Inorganic elements analysed by atomic emission spectroscopy (ICPAES) included Al, Ba, Bo, Ca, Fe, Mg, P, K, Na, Sr, S and Ti. Mass spectroscopy (ICPMS) was used to analyse As, Be, Cd, Cr, Co, Cu, Pb, Li, Mn, Mb, Ni, Se, Ag, Tl, Sn, V and Zn. The bioassays and elemental analyses were carried out on water collected from two tanks per treatment group at the end of the 14-day incubation, as well as duplicate fresh preparations of the treatments dosed at the rates specified in Table 1.
Field Trial
The field trial was carried out in a section of the Macquarie River approximately 35 km downstream of Warren; (-31.561, 147.757) to (-31.436, 147.715). At the time of the study Warren Weir had been temporarily raised in response to severe drought conditions in the region. As a result, the study area downstream had ceased to flow for approximately one month prior to the field trial and become a series of disconnected refuge pools. The TWC and barley straw treatments were selected for field studies as they did not require continuous dosing at the remote study location.
Three replicate pools of 2-5 m depth were assigned to barley straw, TWC, aerator and control treatments. Barley straw was packed into polymer mesh bales and floated in the pools, attached to fallen trees or cinderblock anchors. The pools were dosed at approximately 25 g dry straw weight / m2 of pool surface area. This dose rate was in the lower range of dosage rates reported in the literature (Geiger et al., 2005) to minimise the risk of low dissolved oxygen from decaying straw. Water Cleanser blocks were dosed to the manufacturer’s recommendation of 1 kg per 100 m2 of pool surface area. Each block was attached to a float with nylon rope and placed at regularly spaced intervals along the channel in each pool. For the aeration treatment, two solar bubble plume aerator units (model TSG500B, Solair Group Water Systems) were positioned on the riverbank and connected to ring diffusors that were placed in the deeper channel sections of each pool. The aerator units were operated on an alternating 12-hour timer cycle to allow for continuous aeration while each battery unit was charging.
The 12 study pools were sampled over 10 weeks, from 29/10/2019 to 04/02/2020, with approximately monthly surface grab samples taken for turbidity (HACH turbidimeter), cyanobacteria, nutrients, carbon and fortnightly depth profiles of physical and chemical parameters were measured with an EXO multiparameter sonde (Xylem). Surface grab samples were collected for algal, nutrient and carbon analysis. Nutrient and organic carbon samples were analysed following standard methods (Rice et al., 2017). Algal samples were analysed by microscopic identification and enumeration (Rice et al., 2017). The first round of baseline sampling was collected immediately prior to treatment installation in late October 2019. Monitoring was scheduled to continue until April 2020 but was terminated when the sites were impacted by a series of high flow events from late January.
Statistical analysis
All statistical analyses were performed in R version 3.6.2. Baseline sampling data collected prior to treatment dosing were excluded from all modelling carried out on both laboratory and field datasets.
For the laboratory trial, mixed modelling was used to test the overall effect of treatment type on cyanobacteria counts, physical and chemical variables, nutrients, algal areal standard units and total diatom count (Table 2). Non-linear models were used for variables where gaussian models were a poor fit, as assessed from diagnostic plots of model residuals. Where treatment effects were significant, post-hoc contrasts were run to identify which treatments differed significantly from the control. The effects of treatment and day on Day 1 and Day 14 algal community structure was tested using permutational ANOVA (4999 runs) with post-hoc contrasts.
For the field trial, pools were considered stratified if there was a > 4 mg/L difference between maximum and minimum dissolved oxygen across the depth profile. Principal component analysis (PCA) was used to reduce the environmental variables into two combined water quality variables (PC1 and PC2) for further modelling. The relative effect of predictor variables on cyanobacterial counts were assessed using generalised linear mixed models with a gamma error distribution, including site as a random intercept to account for the repeated measures design. Initial full models for cyanobacterial count were defined with treatment (treat), day, stratification state (strat), environmental PC1 and PC2 variables and a treatment x day interaction as fixed terms (Table 3). Predictors were sequentially dropped from the full models to assess which specific terms contributed to an improvement in model fit, assessed using the Akaike Information Criterion for smaller sample sizes (AICc). Analysis of deviance was used to assess the significance (p<0.01) of individual model terms (Type 2 Wald chi-square tests). The optimum model was defined by selecting only significant terms from the full model and validated by assessing residuals vs fits and normal q-q plots.
Results
Laboratory trial
There was a significant overall effect of treatment on cyanobacteria count (Table 2), which was lower in the barley straw treatment relative to the control (Figure 1). Pairwise comparisons showed that cyanobacterial counts in the Aqua-Cal and barley straw treatments were lower than the control at the Day 5 growth peak (Table 2). However, cyanobacterial counts in the Aqua-Cal treatment were higher than the control at the end of the incubation (Table 2). Cyanobacterial counts in the Diatomix and TWC block treatments were not significantly different from the control at Day 5 or Day 14 (Table 2). The Aqua-Cal and barley straw treatments had significant effects on individual water quality response variables, as summarised in Table 2.
There was a significant effect of treatment (F=2.253, p<0.05) and day (F= 23.636, p<0.05) on algal community structure, with a significant treatment x day interaction (F=1.819, p<0.05). Algal community structure in the treatment groups was similar to the control on Day 1, and was heavily dominated by the genera Scenedesmus, Aphanocapsa and Cyanogranis in all treatment groups. At Day 14 a significant difference was detected between the algal community in the barley straw and control treatments (p<0.05). This difference in community structure was driven by a predominance of green algal taxa in the barley straw tanks, including the Dictyosphaerium, Scenedesmus and Oocystis families. This contrasted with the higher relative abundance of cyanobacterial taxa observed in the control and many of the other treatment group tanks at Day 14.
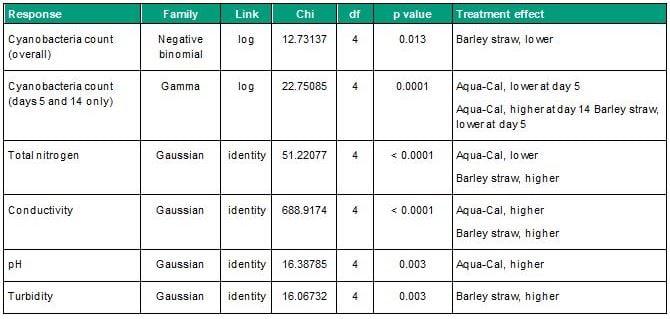
No toxicity to rainbow fish, water fleas or bacteria was detected from either the fresh treatment preparations or the tank water collected at the end of the 14-day incubation. None of the fresh or aged treatments released inorganic constituents or petroleum hydrocarbons at concentrations of ecological concern.
Field trial
Baseline measurements taken on 29/10/2019 show that all pools were stratified at 1-2 m depth prior to treatment application. Aerator pools generally remained mixed from November 2019 to January 2020. Some stratification was visible in aerator pools on some sampling dates when aerators had temporarily stopped working due to falling pool levels. The remaining block, barley straw and control pools remained stratified for most of the study duration, with some periodic mixing during storm events. Concentrations of DOC, TN, TP and NOx generally increased from baseline levels during the study period, whereas ammonia and SRP were more variable and remained close to detection limits (data not shown). The principal components analysis of physical-chemical and nutrient variables produced PC1 and PC2 components that explained 35.9% and 19.8% of total environmental variation respectively.
Algal and cyanobacterial growth was highly variable over time and within treatment groups on individual dates, with initial cyanobacterial cell counts ranging from 0 to ~600 000 cells/mL (Figure 2). Treatment type did not significantly contribute to variation in cyanobacteria counts in the test pools (Figure 2a, Table 3), although a visual improvement in water clarity was noted at one of the three pools treated with TWC. There was no obvious temporal pattern in cyanobacteria count within study pools (Figure 2b). Stratification status was a strong contributor to variation in cyanobacterial counts (Figure 2c, Table 3). Comparison of AICc values shows that the quality of the cyanobacteria count models improved when the treatment, day and treatment x day interaction terms were excluded (Table 3). This indicates that these factors explain little additional variation in the count data than the environmental variables PC1, PC2 and stratification, which was selected as the optimum model (Table 3). Inspection of diagnostic plots showed this model was a suitable fit and did not violate the assumption of equal variance of the residuals.

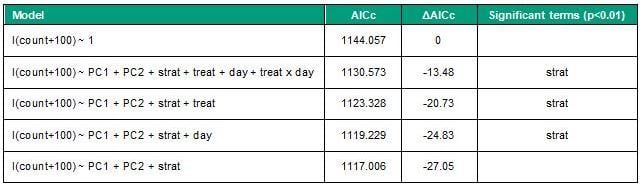
Discussion
The barley straw was the only treatment to show a significant overall effect on cyanobacterial growth in the laboratory trial, which occurred alongside a shift in algal community structure from a cyanobacterial to a green algae dominated assemblage. However, the barley straw treatment also significantly increased conductivity, turbidity and total nitrogen concentration relative to the control. These water quality effects may be of concern for drinking water treatment. It was expected that the rotting barley straw may have adversely impacted dissolved oxygen. A reduction in dissolved oxygen was observed in some individual barley straw laboratory tanks but not others, and where a reduction did occur it was to a similar concentration as the control. This suggests that reduced dissolved oxygen was a result of containment in the laboratory rather than the decomposition of the barley straw. For the field trial, the barley straw dose rate was approximately halved to reduce the risk of low oxygen caused by straw rotting in high summer temperatures. This lower dose may have reduced the treatment effectiveness in the field trial. It is also possible that the field trial was too short for a potential effect of the barley straw to be detectable, as it may take months for a cyanobacterial response to be apparent in the field (Geiger et al., 2005, Ó hUallacháin and Fenton, 2010).
The TWC block treatment did not have a statistically signficant effect on cyanobacterial count in the laboratory or field trials, nor did it significantly affect any of the measured water quality or treatability parameters in the laboratory trial. An effect of the TWC block should have been detected within the 10-week field trial, as the product requires four to ten weeks to become effective. The lack of treatment effect may also have been due to an absence of the Bacillus spp. bacteria that are claimed to mediate the anti-cyanobacterial effects of this product. If this were the case, this implies that this treatment would not be effective if deployed in environments where these bacteria are not present. Alternatively, additional supplementation with live Bacillus spp. may be required for the treatment to be effective in systems where these bacteria are not naturally occurring.
In the laboratory study, cyanobacterial count in the Aqua-Cal treatment was lower than the control at Day 5 but higher at Day 14, resulting in no statistically significant effect of the Aqua-Cal treatment overall. At Day 14, there was a notably stronger presence of the cyanobacteria Pseudanabaena in the Aqua-Cal treatment that was not evident in the other treatments, and this may have contributed to the increased total cyanobacterial growth at Day 14. Total nitrogen concentration was significantly lower in the Aqua-Cal treatment than the control treatment, which is consistent with the treatment’s purported mode of action of removing nutrients from the water column. Aqua-Cal treatment also significantly increased conductivity and pH, which may be of concern in drinking water storages.
The Diatomix treatment had no statistically significant effect on cyanobacterial growth or water quality parameters in the laboratory trial. There was also no difference in total diatom count between Diatomix and control treatments at any stage of the incubation. The Diatomix product is intended for use in water where there is excess of nitrogen and phosphorus relative to micronutrients. This nutrient balance is unlikely to occur in Lake Prospect in winter when the water for the laboratory trial was collected. If this is the case then future trials or application of the Diatomix treatment would be most effective in systems with higher nitrogen and phosphorus concentrations, which are more likely to be micronutrient limited.
In the field trial, the stratification state of the pools was the strongest predictor of cyanobacterial count, while treatment type was the least significant predictor. This finding suggests that treatment effectiveness may be reduced or overridden in situations where environmental factors such as stratification strongly promote cyanobacterial growth. In the field trial, pools with aerators were destratified for most of the study but sometimes became stratified. The most likely cause for this is that some of the diffusers temporarily stopped working as the water level in the pools dropped and changed the head pressure balance between the two air lines attached to each aerator. In addition, individual pools in the control and other treatment groups were naturally mixed on some sampling occasions. The occurrence of stratification and mixing in pools across all treatment groups may explain why the modelling identified stratification state but not the aeration treatment as a significant predictor of cyanobacterial response. Taken together, these results still show that destratification is an effective cyanobacterial control treatment.
There is a high likelihood that nutrient limitation in the experimental tanks affected cyanobacterial and algal growth dynamics in the later part of the laboratory incubation. For this reason, the conclusions of this study have explicitly considered individual treatment effects at the Day 5 growth peak, as well as any evidence to support the claimed mode of action of each treatment, even if this did not translate into a detectable effect on cyanobacterial growth in this study. Furthermore, the Microcystis inoculum used in the laboratory study showed low rates of survival after transfer to the experimental tanks. Follow-up investigations (data not shown) found that the nutrients supplied were insufficient to support Microcystis growth, although treatment effects could still be assessed using total cyanobacterial cell count. As with any laboratory study, containment effects must be considered when making field-scale inferences from the findings. In the field study, a higher level of replication may have been required to detect treatment effects over background environmental variation given the high variability in cyanobacterial growth observed within each treatment group.
Conclusions
These trials assessed the effectiveness of cyanobacteria treatment products in a 2-week laboratory study and a 10-week field study. The barley straw treatment significantly limited cyanobacteria growth in the laboratory trial, but not in the field trial. The trials did not provide conclusive evidence to show that Aqua-Cal+™, Diatomix and The Water Cleanser block treatments were effective in limiting cyanobacterial growth, or that barley straw was effective when deployed in the field. No ecotoxicological effects or contaminant release were found from any of the tested treatment products. Stratification state strongly influenced cyanobacterial growth in the field trial, which supports the continued use of destratification by aeration for cyanobacteria management. Any future field trials should run for at least three months and have higher replication to demonstrate the effectiveness of the tested cyanobacteria treatment products. This study produced several learnings that may be applied to guide the design of any future trials.
About the authors
Ann-Marie Rohlfs | Ann-Marie Rohlfs is a Water Quality Scientist at WaterNSW. Ann-Marie currently leads projects to incorporate the latest science and technology into source water catchment and water quality protection. Her work has focussed on monitoring catchment health and infrastructure upgrades and managing water quality impacts of extreme climatic events. Ann-Marie completed her PhD in freshwater ecology, assessing environmental flows in the rehabilitation of the Snowy River.
Joe Pera | Joe Pera is a Water Quality Scientist at WaterNSW. His background is in freshwater ecology, water quality and mechanical engineering. Joe is currently completing a PhD focused on the effects of Carp on ecosystems and impacts on water quality from decaying fish post virus release. He has also worked in carp management and monitoring improvements in water quality from wastewater treatment plant upgrades.
Alec Davie | Alec Davie is a Water Quality Scientist at WaterNSW. His main interests include freshwater ecology and protecting water quality. Alec’s PhD focused on the effects of flow on benthic assemblages in the Severn River, NSW. Before this he completed his Honours investigating the effects of nutrients on phytoplankton blooms in the mid-Hunter River NSW.
References
BALL, A. S., WILLIAMS, M., VINCENT, D. & ROBINSON, J. 2001. Algal growth control by a barley straw extract. Bioresource Technology, 77, 177-181.
BARRETT, P. R. F., LITTLEJOHN, J. W. & CURNOW, J. 1999. Long-term algal control in a reservoir using barley straw. Hydrobiologia, 415, 309-313.
EVERALL, N. C. & LEES, D. R. 1997. The identification and significance of chemicals released from decomposing barley straw during reservoir algal control. Water Research, 31, 614-620.
GEIGER, S., HENRY, E., HAYES, P. & HAGGARD, K. 2005. How barley straw may work to suppress algal growth. Portland: Aquatic Scientific Resources.
HORN, H. & UHLMANN, D. 1995. Competitive growth of blue-greens and diatoms (Fragilaria) in the Saidenbach Reservoir, saxony. Water Science and Technology, 32, 77-88.
Ó HUALLACHÁIN, D. & FENTON, O. 2010. Barley (Hordeum vulgare)-induced growth inhibition of algae: a review. Journal of Applied Phycology, 22, 651-658.
PATRICK, R., CRUM, B. & COLES, J. 1969. Temperature and manganese as determining factors in the presence of diatom or blue-green algal floras in streams. Proceedings of the National Academy of Sciences of the United States of America, 64, 472-478.
RICE, W., BAIRD, R. B. & EATON, A. D. (eds.) 2017. Standard Methods for the Examination of Water and Wastewater: American Public Health Association, American Water Works Association, Water Environment Federation.
SIGEE, D. C., GLENN, R., ANDREWS, M. J., BELLINGER, E. G., BUTLER, R. D., EPTON, H. A. S. & HENDRY, R. D. 1999. Biological control of cyanobacteria: principles and possibilities. Hydrobiologia, 395, 161-172.
WRIGHT, S. J. L. & THOMPSON, R. J. 1985. Bacillus volatiles antagonize cyanobacteria. FEMS Microbiology Letters, 30, 263-267.
WU, Q., BISHOP, P. L., KEENER, T. C., STALLARD, J. & STILE, L. 2001. Sludge digestion enhancement and nutrient removal from anaerobic supernatant by Mg(OH)2 application. Water Sci Technol, 44, 161-6.
YU, J., KONG, Y., GAO, S., MIAO, L., ZOU, P., XU, B., ZENG, C. & ZHANG, X. 2015. Bacillus amyloliquefaciens T1 as a potential control agent for cyanobacteria. Journal of Applied Phycology, 27, 1213-1221.